Research in the Radiation Research and Detector Development R2D2 Laboratory
Brief outline of some of the research:
1. Fission:
Working with LANL on prompt neutron and gamma numbers and spectra from Pu fission. Currently analyzing 242Pu spontanous fission data taken with the Chi-Nu array at LANSCE.
Prior work, just finishing data analysis:
Fragment spectrometer for improved yield data
- Collaboration with LANL
- Gathering mass data
- Current detector development focus on Z determination through fragment range in ionization chamber
2. Machine Learning
- ML for indentification of threats in radiation spectra
- ML combined with neuromorphic computing for low power spectral identification for independent monitoring
- Prior DTRA project in collaboration with electrical engineering and Portland State University
3. Muon Imaging:
- Muon imaging with a focus on used fuel storage casks
- Gas detectors on opposite sides of cask to measure position and angle of muons to determine scattering intensity (proton number dependent) vs position
- Projection imaging for 1D intensity image. Combining to form tomographic images
- Useful to verify contents to detect diversion of special nuclear materials
4. Detector development
Perovskite based radiation detector
- Room temperature detection
- Collaboration with UNLV, tests at LANL
- Growth and characterization of material for alpha and gamma ray spectroscopy
Room temperature semiconductor detector based on AlSb
- Predicted better properties than CZT, but difficult to grow
- Able to grow by molecular beam epitaxy with good results
- Able to grow directly on Si for CMOS integrated detection
- This means low cost, low power, very sturdy and very deployable detectors
5. Many past projects include optical evaluation of crystals to neutron damage as a gamma blind neutron dosimeter, fission simulations, x-ray image tracking for examining moving parts within components, environmental analysis based on examination of uranium transport in soils, and many more.
There are many research topics important to the nuclear engineering community within radiation detection but a few strong motivating themes dominate: nuclear safeguards and nonproliferation, and improved data, especially fission data. I built a multidisciplinary research program on radiation detection and detector development at the University of New Mexico with an emphasis on addressing these needs. My goal is to continue to conduct research in areas of high importance to the nuclear engineering community while supporting the overall mission of UNM Nuclear Engineering.
Below I highlight my research focus areas, beginning with addressing needs in fission data, making these data useful through simulations, and then new detector development work that can find applications in materials control and accountability for safeguards, and for detection out in the field.
1. Fission Data:
Prompt neutron and gamma numbers and energy spectra in collaboration with Chi-Nu group at LANL:
Currently analyzing 242Pu spontaneous fission emission, data taken at LANL on Chi-Nu array.
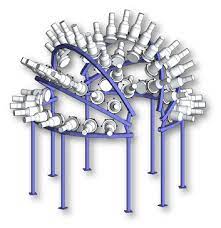
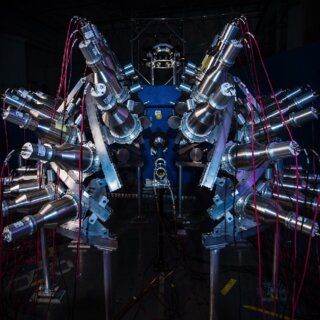
Fission Spectrometer:

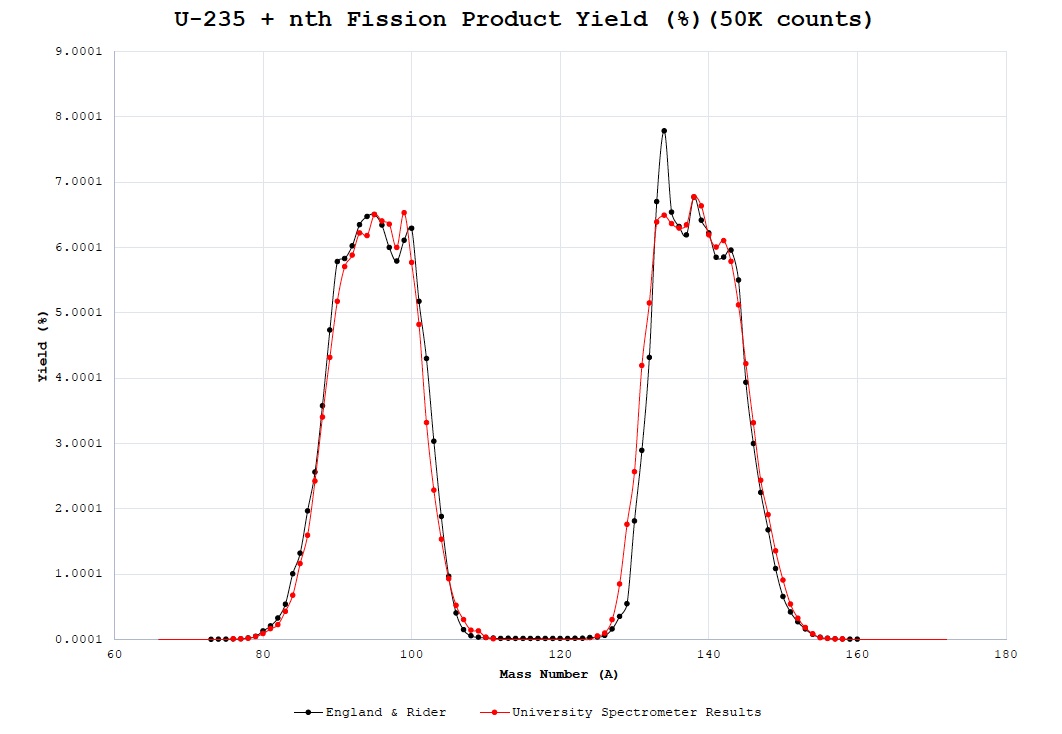
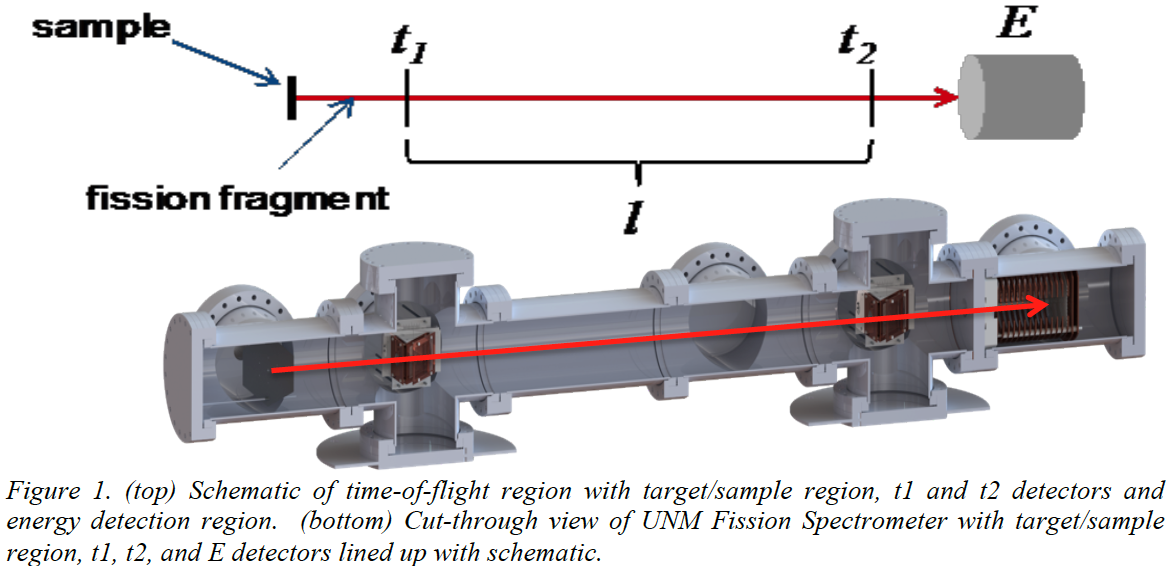
UNM Fission Fragment Spectrometer and 235U mass data
We have built (and continue improving) at UNM a spectrometer for high resolution data on fission mass distributions. We are in collaboration with researchers at the Los Alamos Neutron Science CEnter (LANSCE) at LANL on the SPIDER project (SPectrometer for Ion DEtermination in fission Research), to measure fission fragments, particle by particle, over a range of incident neutron energies over a range of fission targets. We were originally funded through a contract with Los Alamos and then changed over to a DOE-NEUP award.
See summary at: http://news.unm.edu/news/unm-professor-leads-team-exploring-nuclear-fission
The spectrometers (at both UNM and LANL) are based on time-of-flight (TOF) fragment velocity measurements and ionization chamber (IC) energy measurements, with the data correlated particle-by-particle, which itself is very involved. From E and v for each particle, following E=1/2 mv2, we extract fragment mass. A mass distribution plot from the UNM spectrometer from correlating v and E is presented in the figure (before E loss corrections). Some of our TOF work is presented in reference [3] and more papers are in preparation.
The full collaboration with LANL is focused on building high resolution, good geometric efficiency spectrometers and gathering fission fragment data. LANL has considerable resources and personnel for their system development, and so the UNM effort is focused on prototyping detectors and methods to increase capabilities for both UNM and LANL, and in the process we make our own full spectrometer system and gather independent data sets. For example, we designed and tested the UNM ionization chamber that LANL based their IC design on. We built a full UNM-SPIDER detector and tested it with both alpha and fission sources, and with neutron beams on 235U at LANSCE.
By examining depth of penetration in the gas in the ionization chamber, using the IC as a time projection chamber, we are able to extract information on the charge state of the fragment. My group at UNM is currently focused on using an active cathode method in the ionization chamber to extract proton number (Z) information, beyond what the LANL group is currently doing with their focus on mass (A). Our capabilities thus will give us Z in addition to A (and thus we measure N). Moving forward to measure both fragments simultaneously in binary fission we will measure fission TKE and, almost directly, neutron multiplicity, both extremely important for theory. We are in the process of producing strong and novel data sets to deliver to theorists and the nuclear community.
A report is available at https://neup.inl.gov/SiteAssets/Final%20%20Reports/FY%202013/13-4815%20NEUP%20Final%20Report.pdf
The takeaway: We are collecting quasiprompt (50-100 ns) fission product data, with E, A, Z correlated particle-by-particle.
See Richard Blakeley, Development of The University of New Mexico Spectrometer for High-Resolution Fission Product Yield Data
2 Machine Learning
We're doing good and interesting things. I just need to update the website to show you.
3 Muon imaging
Another research example is the use of muons for imaging, with projection images of used fuel storage casks
see Daniel Poulson, Interrogation of Spent Nuclear Fuel Casks Using Cosmic-Ray Muon Computed Tomography
and with proof of principle experiments such as we ran on the UNM reactor.
see John Perry, Advanced Applications Of Cosmic-Ray Muon Radiography
4 Detector development
Room temperature radiation detection using perovskites:
Working with UNLV and LANL to produce, characterize the material, and test with alpha and gamma ray radiation. More to put here soon.
Room temperature semiconductor detectors based on AlSb:
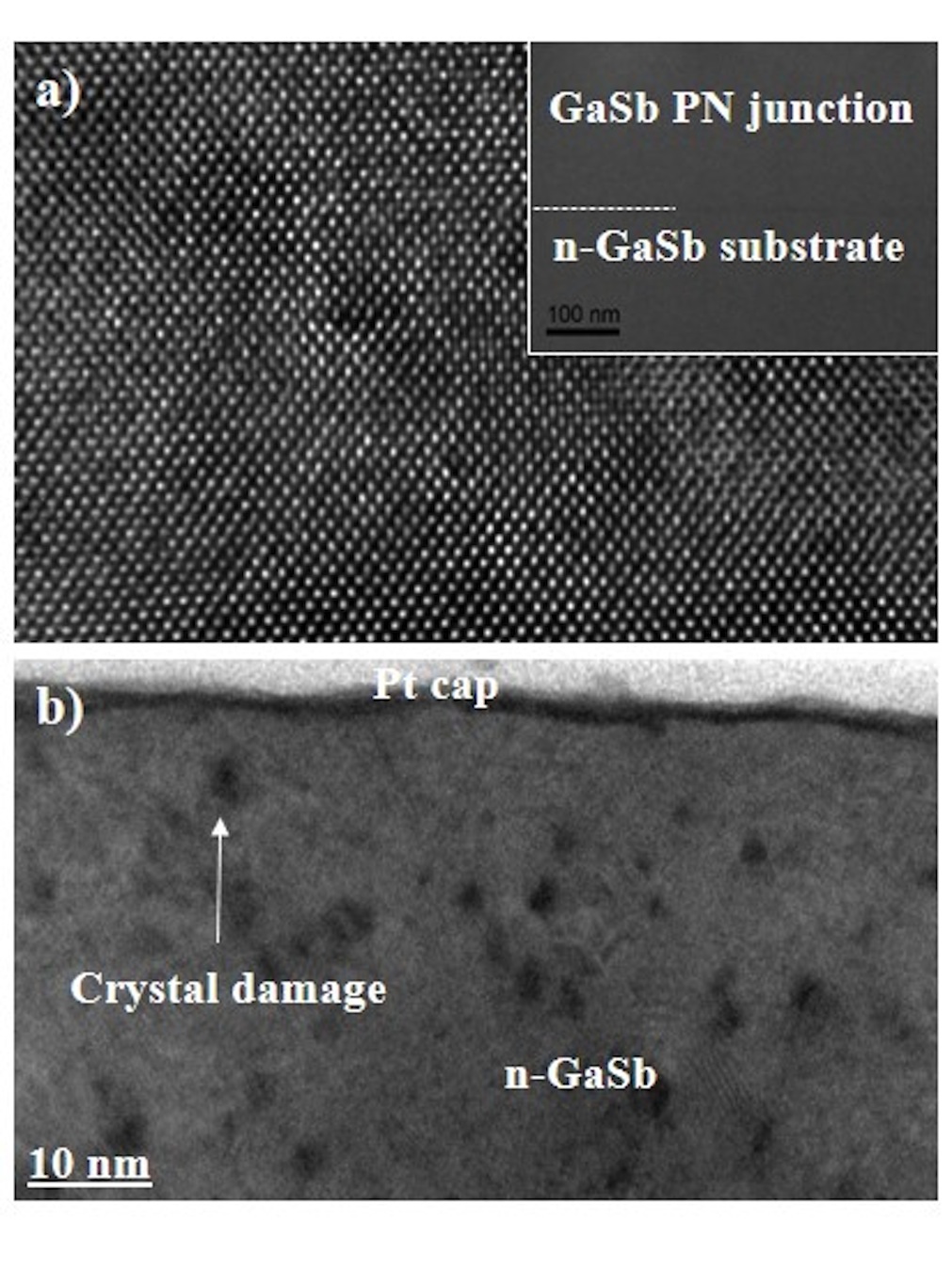
Probe station for radiation characterization with AlSb semiconductor samples and TEM profile image of different GaSb diode structures.
In nonproliferation, interdiction of SNM smuggling, or identification of radioactive threats - all important applications of nuclear science - gamma ray spectroscopy is an important mode of identification and quantification. Unfortunately, typical detectors such as NaI or other scintillators have low resolution, while high-resolution semiconductor detectors such as Ge are difficult to field.
There has been a lot of research on room temperature semiconductor detectors, and CdZnTe (CZT) has been of great interest. Due to poor hole mobility in CZT the induced signal pulse varies greatly depending on position of radiation interaction relative to the electrodes. This is one reason usable CZT crystals are on the mm scale, greatly limiting efficiency. Though tricks have been implemented using multiple electrodes, this is still a materials deficiency. AlSb had been proposed as a very strong candidate for room temperature detectors, with electron and hole properties predicted to be similar and near that for electrons in CZT [8], which would give a more symmetric detector response allowing larger theoretical volumes. As a dual carrier detector, this also improves statistics and therefore resolution over CZT. At issue is how to grow pure and defect free materials to test the theorized properties. LBNL was researching the material properties, improving methods in growing bulk crystals of AlSb, but Al oxides rapidly and Sb reacts even with the growth crucibles and high enough quality material was never obtained.
The expected characteristics of AlSb are too tantalizing, and there are other techniques to achieve high quality growth. Teaming with Prof. G. Balakrishnan in Electrical Engineering, an expert in epitaxial growth of antimonides, we have been pursuing a campaign to grow high purity, low defect AlSb for radiation detection an atom layer at a time using molecular beam epitaxy (MBE). We have ideas to scale up to thicker detectors. MBE growth allows much more control over the crystal than bulk techniques, and we are already seeing success with thin films. To distinguish roles in this, Prof. Balakrishnan's students grow the materials, and my student does materials electronic testing (Hall measurement, mobility, resistivity, carrier concentration, and soon carrier lifetime) and characterizing the radiation response, which feeds in to the next iteration of growth and material doping. Our research strengths are very complementary in this collaboration to make room temperature detectors.
We have grown and tested thin film GaSb [9], which has much more ideal purity and low defect properties. We have grown and tested several samples of AlSb, manuscript in preparation, and already used these for alpha spectroscopy, and results are guiding specifics of current material growth. If we prove the predicted properties then this opens a new material for room temperature detection and spectroscopy.
See Erin Vaughan, Thin film AlSb carrier transport properties and room temperature radiation response
References
Updating with website
g.s. denotes my graduate students
1. http://www.mcs.anl.gov/events/workshops/nprcsafc/Report_FINAL.pdf
2. Fission fragment isomers populated via 6Li + 232Th, J.J. Ressler, J.A. Caggiano, C.J. Francy, P.N. Peplowski, J.M. Allmond, C.W. Beausang, L.A. Bernstein, D.L. Bleuel, J.T. Burke, P. Fallon, A.A. Hecht, D.V. Jordan, S.R. Lesher, M.A. McMahan, T.S. Palmer, L. Phair, N.D. Scielzo, P.G. Swearingen, G.A. Warren, M. Wiedeking, Phys. Rev. C, 014301 (2010).
3. Development of position-sensitive time-of-flight spectrometer for fission fragment research, C.W. Arnold, F. Tovesson, K. Meierbachtol, T. Bredeweg, M. Jandel, H.J. Jorgenson, A. Laptev, G. Rusev, D.W. Shields, M. White, R.E. Blakeley g.s., D.M. Mader g.s., A.A. Hecht, Nuc. Instr. Meth. A 764, 53 (2014); doi:10.1016/j.nima.2014.07.001
4. Comparison of Geant4 and MCNP6/CINDER for use in Delayed Fission Radiation Simulation, A.A. Hecht, R.E. Blakeley g.s., W.J. Marting.s., E. Leonardg.s., Annals of Nuclear Energy 69, 134 (2014); doi:10.1016/j.anucene.2014.02.004
5. Dual neutral particle induced transmutation in CINDER2008, W.J. Martin g.s., C.R.E. de Oliveira, A.A. Hecht, Nucl. Instr. Meth. A 767, 163 (2014); doi:10.1016/j.nima.2014.08.048
6. Reactor fuel depletion benchmark of TINDER, W.J. Marting.s., C.R.E. de Oliveira, A.A. Hecht, Annals of Nuclear Energy 73, 547 (2014); doi:10.1016/j.anucene.2014.04.036
8. Room-Temperature Replacement for Ge Detectors-Are We There Yet? P.N. Luke, M. Amman, IEEE Trans. Nuc. Sci. 54, 834 (2007); doi:10.1109/TNS.2007.903184
9. Thin Film Gallium Antimonide for Room Temperature Radiation Detection, Erin I. Vaughan g.s., Nassim Rahimi, Ganesh Balakrishnan, Adam Hecht*, Submitted to Journal of Electronic Materials
10. Novel Techniques for High Precision Refractive Index Measurements, and Application to Assessing Neutron Damage and Dose in Crystals, K. Masuda, E.I. Vaughan g.s., L. Arissian, J.P Hendrie, J. Cole g.s. g.s., J.-C. Diels, A.A Hecht*, Nuc. Instr. Meth. A (2014), accepted 11/11/14. http://dx.doi.org/10.1016/j.nima.2014.11.031
11. Radiolytic yield of ozone in air for low dose neutron and x-ray/gamma-ray radiation, J. Cole g.s., S. Su g.s., R.E. Blakeley g.s., P. Koonath, A.A. Hecht, Radiation Physics and Chemistry 106, 95 (2015); doi: 10.1016/j.radphyschem.2014.06.008
12. Imaging a nuclear reactor using cosmic ray muons, J. Perry g.s., M. Azzouz, J. Bacon, K. Borozdin, E. Chen, J. Fabritius II, E. Milner, H. Miyadera, C. Morris, J. Roybal, Z. Wang, B. Busch, K. Carpenter, A.A. Hecht, K. Masuda, C. Spore, N. Toleman, D. Aberle, Z. Lukić, J. Appl. Phys. 113, 184909 (2013); doi: 10.1063/1.4804660
13. Identifying Nuclear Materials Using Tagged Muons, C. L. Morris, J. D. Bacon, K. Borodzin, J. M. Durham, J. M. Fabritius II, E. Guardincerri, A. Hecht, E. C. Milner, H. Miyadera, J.O. Perry g.s., D. Poulson g.s. , Submitted to Nucl. Instr. Meth. A
14. Ground water contamination with 238U, 234U, 235U, 226Ra and 210Pb from past uranium mining: Cove Wash, Arizona, K. da Cunha, H. Henderson, B.M. Thomson, A.A. Hecht, Environmental Geochemistry and Health (2013); doi:10.1007/s10653-013-9575-2